Ultrapure Water for HPLC Analysis
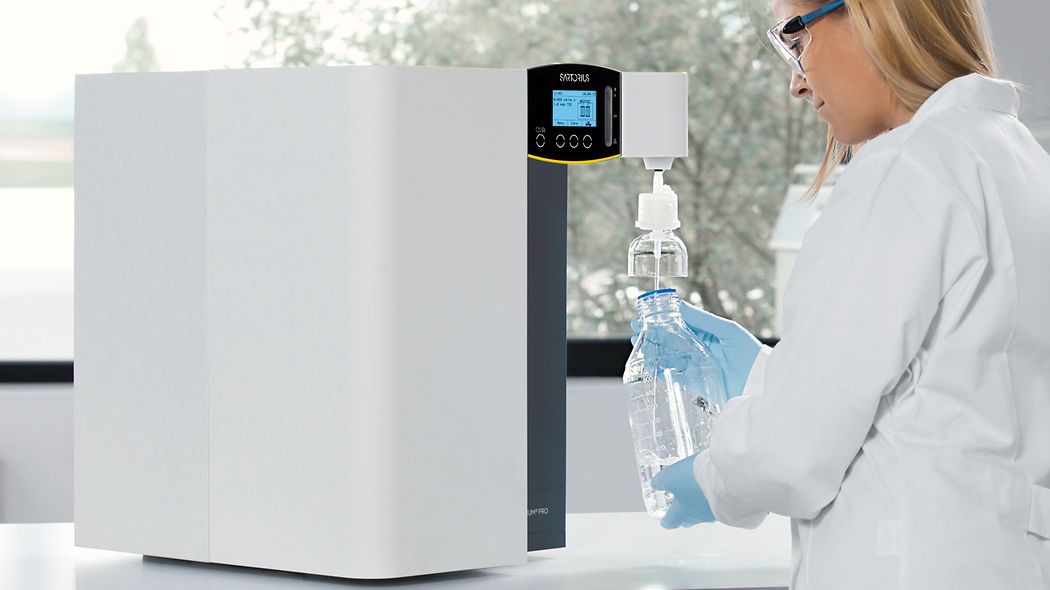
Introduction
HPLC (High-Performance Liquid Chromatography) is an analytical procedure for the separation, identification, and quantification of substances using liquid chromatography. The origins of HPLC date back to the 1960s. Thanks to improvements in column materials and equipment, it has been known as High-Performance Liquid Chromatography since the late 1970s. In HPLC, the mixture to be separated is transferred to a column with a solvent (eluant) or a mixture of solvents (mobile phase) via an injector and a pump. The column is a tube, mostly made of stainless steel, filled with the so-called stationary phase (see also Fig. 1). The stationary phase usually consists of porous silica gel or polymer particles with chemical ligands attached to their surface. These ligands are responsible for the selective interactions between the analytes and the stationary phase, which are necessary for effective chromatographic separation. Depending on the sample and the stationary phase, the separation mechanisms involved include adsorption by Van der Waals forces, ion exchange, size exclusion, etc.
The substances from a sample are retained in the column packing material for different periods of time, and therefore elute from the column after different retention times. The individual components of the sample are then recorded by a detector and evaluated by a computer. The result is a chromatogram (Fig. 1, 5, 6). The number of peaks corresponds to the number of components separated in the sample, and the area is proportional to the concentration of those separated components (according to Kromidas 2000).
Among the typical applications of HPLC is the analysis of sugars. This was conducted within the framework of several tests aimed at characterizing the quality of membranes. On the one hand, the membranes were tested for their ability to remove sugar molecules, and on the other hand, the activity of enzyme-immobilized membranes was determined. For this purpose, sugars such as raffinose, glucose, and fructose were tested. These types of sugars can be specifically detected using enzymatic methods such as the GOD|POD assay for glucose or spectroscopic methods, such as the determination of fructose according to Dische & Borenfreund.
In advanced analysis, sugar is now often tested by thin-layer chromatography (TLC), gas chromatography (GC), and high-performance liquid chromatography (HPLC). These methods are especially used when mixtures containing various types of sugar need to be separated. In HPLC, as described here, the eluate must have an especially high physical and chemical purity and should not contain suspended mechanical particles or dissolved substances that could be released by the column at a later time, thereby generating a signal. The quality of a solvent is often decisive for the reliability of an analytical HPLC run. The presence of trace contaminants during gradient elution can result in “ghost peaks.” These trace substances accumulate in the column during an analytical run and are gradually released when the eluant is subsequently changed. The water used as the eluate must be free of microorganisms. For this, substances can be added to prevent the growth of microbes and algae in the solvent mixture, such as copper salts or sodium azide. When doing this, the recommendations of the column manufacturer should be followed, as using incorrect additives can cause irreversible damage to the column.
Deionized or distilled water still contains considerable amounts of organic substances, which can cause ghost peaks. Contaminated solvents can lead to the accumulation of deposits in the stationary phase, resulting in column blockage, which would manifest as an increase in pressure and a change in sample run time.
Use of Arium® Pro VF to Purify Water for Use as Eluant
The special quality water required for HPLC can be purchased from various manufacturers or produced on-site for on-demand use, utilizing a water purification system such as the Arium® Pro VF system. The following describes the tests conducted for the separation of sugar mixtures in which ultrapure water produced by the Arium® Pro VF was used as the mobile phase (eluant).
Description of the Arium® Pro VF Ultrapure Water System
The Arium® Pro VF system (Fig. 2) was designed to produce ultrapure water from pre-treated drinking water, removing contaminants still present in that water. The production of ultrapure water requires continuous recirculation and a constant flow rate, which is achieved using an incorporated pump system with controlled pressure. The conductivity of the water is measured at the inlet of the feed water and directly at the downstream outlet (product water outlet). The Arium® Pro VF system used in the studies described in this article (a predecessor model with the same technical specifications as the currently redesigned system shown on the following page) operates with two different cartridges. These are filled with a special active carbon adsorbent and mixed bed ion exchange resins to provide ultrapure water with low TOC content. Additionally, the system has an integrated UV lamp that has an oxidative effect at wavelengths of 185|254 nm. Moreover, the Arium® Pro VF ultrapure water system features an integrated ultrafiltration module that acts as a cross-flow filter. The ultrafiltration membrane incorporated into this filter retains colloids, microorganisms, endotoxins, RNA, and DNA. A final 0.2 µm filter installed at the water outlet serves to remove particulates and bacteria during the dispensing of the produced ultrapure water. The process the unit employs to purify the water is illustrated in Figure 3 (flow diagram of Arium® Pro VF).
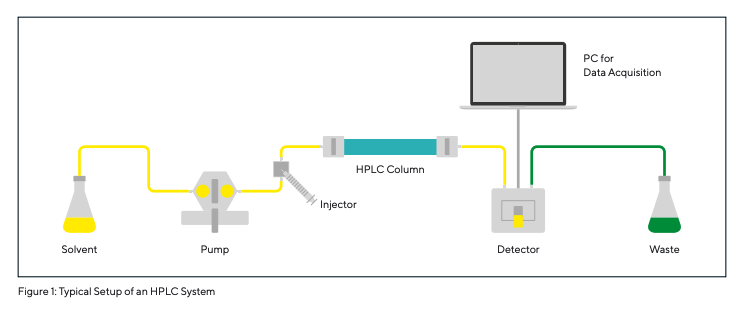
Materials and Method
The samples were analyzed using an Agilent 1200 Series HPLC system (Fig. 4 and Table 1) with a Na+ 8% Rezex carbohydrate HPLC column provided by Phenomenex. This column is filled with a polystyrene-divinylbenzene copolymer modified by sodium sulfonate groups and utilizes an ion exclusion mechanism. This means that the analytes are separated based on different ionic interactions. Due to the sulfonate groups on the surface of this column packing material, the pores have a negative charge. As a result, negatively charged molecules cannot penetrate the pores of the material, causing them to elute earlier. This ion exclusion mechanism is based on the Gibbs-Donnan equilibrium that governs the behavior of ions near a membrane. The analytes that manage to penetrate the pores of the membrane are subsequently separated based on steric differences, as well as hydrophobic and polar interactions with the functional groups on the surface of the stationary phase. For more details on this separation mechanism, see 7.
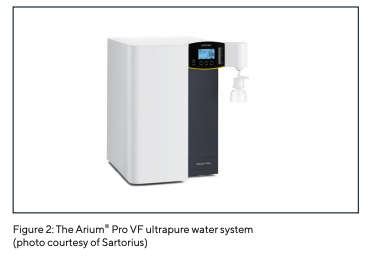
The retention times for various types of sugar are determined by the absorbance of the refractive index (RI) signal. This RI signal is expressed as a dimensionless number in Nano Refractive Index Units (nRIU) and indicates the difference between the refractive index of the sample in the sample cell and the mobile phase in the reference cell. The ultrapure water produced by the Arium® Pro VF system was used as the mobile phase. To degasify the eluate in the HPLC system, this ultrapure water was vacuum-filtered through a disposable Sartolab BT 500 Bottle Top unit equipped with a 0.2 µm membrane (Sartorius Sartolab BT 180C5).
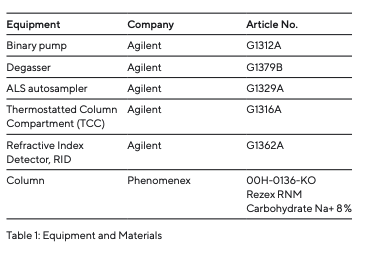
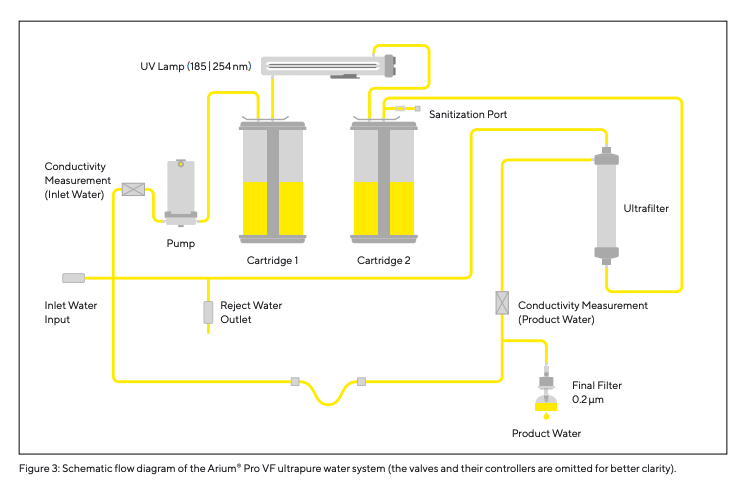
Procedure for HPLC Analysis
To prepare for the analytical runs, the Rezex column was heated to 75°C in the column compartment (heater) and washed overnight with ultrapure Arium® Pro VF water at 0.6 mL/min. The optical unit of the RI detector was heated to 35°C. The samples to be analyzed were prepared using ultrapure Arium® Pro VF water and pre-filtered through a 0.2 µm syringe filter unit (Sartorius Minisart® RC4, no. 17822). The samples were analyzed using HPLC according to the parameters defined by an HPLC method (Table 2).
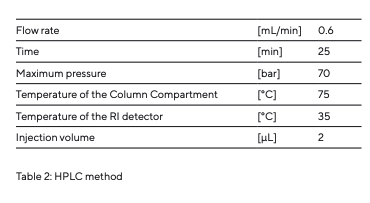
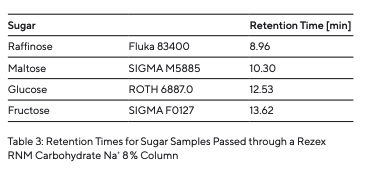
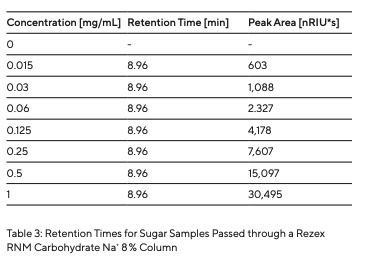
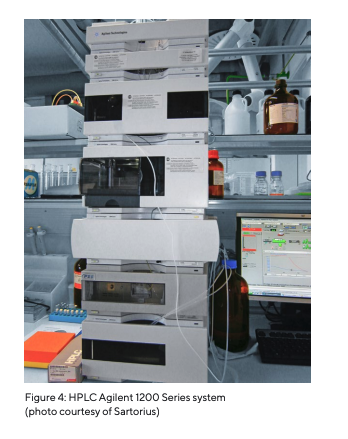
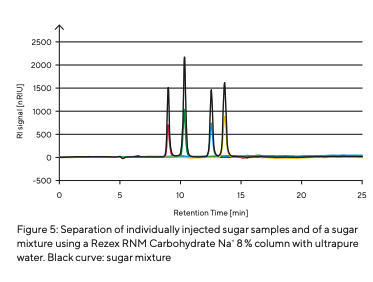
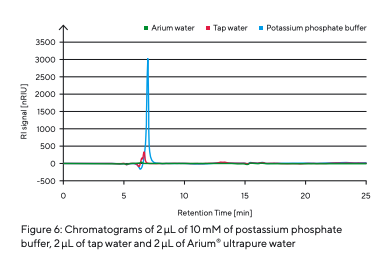
Results
To determine the retention times of different types of sugar (Table 3), they were prepared and injected individually (Fig. 5). As different sugars interact with the stationary phase to varying degrees, specific retention times are recorded by the RI detector as each sugar passes through the column. After determining the different types of sugar, a sugar mixture was prepared and separated (Fig. 5). The individual sugar components were separated from each other. The peaks of different retention times could be attributed to the individual sugar samples analyzed. The effect of contaminants or the influence of salts was simulated by injecting a potassium phosphate buffer and tap water (Fig. 6).
The injection of tap water with a conductivity of 265 µS/cm and the potassium phosphate buffer with a conductivity of 1,700 µS/cm showed clear signals and could therefore be clearly identified as contamination.
Ions with multiple charges are especially prone to binding to sulfonate groups. This alters the dissociation equilibrium and can affect the retention time of a specific sugar. For this reason, the mobile phase must be free of salts and other contaminants to perform reliable HPLC analyses with stable retention times and avoid ghost peaks. The ultrapure Arium® water used in this analysis has a conductivity of 0.055 µS/cm and is virtually free of interfering contaminants, which is reflected as a flat baseline without peaks (see the green baseline in Fig. 6). The column pressure during the analytical runs remained consistently at 23 bar (~334 psi).
This indicates that no deposits were formed in the column. Blank runs at the beginning and end showed no change; i.e., there were no contaminants in the mobile phase. Standard series with different concentrations were analyzed to determine reproducibility and detection limit. Raffinose is given as an example of these conducted series. The retention times and areas of the peaks were recorded and are listed in Table 4. The consistently obtained retention times show excellent reproducibility. The standard series of raffinose presents a linear correlation of the peak areas (R2 = 0.998) (Fig. 7).
Conclusion
The results show that the ultrapure water produced by the Arium® Pro VF system is suitable for use as a mobile phase in HPLC analysis. The analysis of sugar mixtures demonstrated that the column remained free of contamination, as indicated by the flat baseline of the RI detector. Moreover, the ultrapure Arium® water meets the requirements for use as a solvent in analytical methods and ensures consistent and reliable HPLC results.
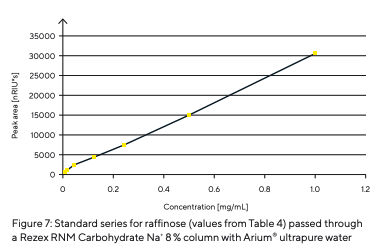