Scaling up E. coli Culture for an Optimized Feeding Strategy in Fed-batch Mode Using Exponential Feeding
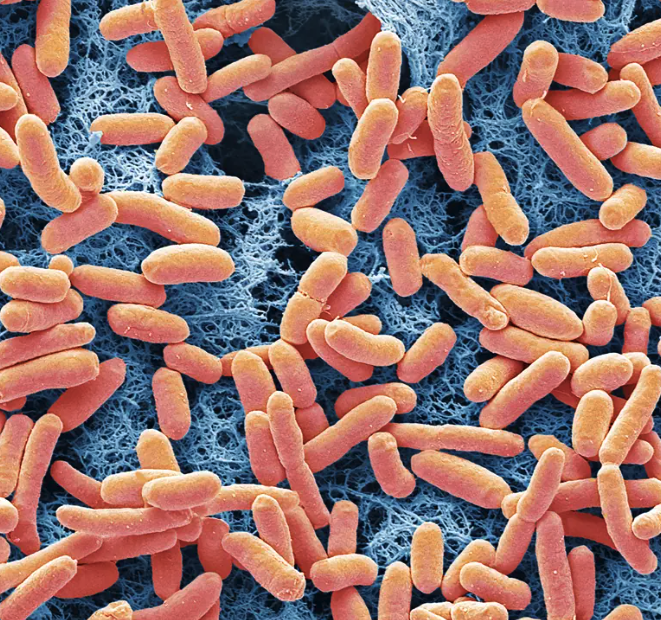
Escherichia coli is one of the most popular expression systems for producing recombinant proteins. In this study, a brief protocol was carried out to apply a fed-batch mode at a laboratory scale with E. coli and scale it up to 30 liters. High cell density of E. coli growth was achieved using fed-batch mode in a Bionet F1 bench-top bioreactor, after batch mode characterization, using an online optical density probe to automatically record growth. A specific growth rate of 0.46h⁻¹ was obtained, resulting in a 7-fold increase in biomass concentration with the fed-batch strategy, compared to the alternative batch mode. The developed process at laboratory scale was scaled up by a factor of 10, under the criterion of maintaining constant volumetric oxygen transfer coefficients (kLa), in a Bionet F2-30A pilot-scale bioreactor.
1. INTRODUCTION
Protein expression in E. coli bacteria has been the most popular expression system for over two decades for producing recombinant proteins due to its enhanced ability to quickly reach high cell densities in cost-effective culture media, with short cultivation time, easy genetic manipulation, low media cost, and approved regulatory status for human and animal applications.
For aerobic fermentation scaling, the effect of gas-liquid mass transfer is the most significant factor. Therefore, aerobic fermentation scaling is often performed based on maintaining a constant volumetric mass transfer coefficient (kLa) across all scales.
The success of the scaling process is generally confirmed by experimental results showing limited differences between small and large-scale fermentations performed under the same oxygen transfer rate.
In this work, the growth of an E. coli strain was characterized at the laboratory scale using the Bionet F1 bioreactor through online optical density measurements to establish the optimal substrate feeding rate in fed-batch mode during the exponential phase. With the obtained data, the process was scaled up to a 30-liter fermenter, using the Bionet F2-30A bioreactor and applying a scaling ratio of approximately 1:10.
MATERIALS AND METHODS
Equipment
- Bioreactors/Fermenters: Table 1 provides a complete description of the equipment used:
- Bionet F1-3MB Bench-top Bioreactor (Fig. 1) with ROSITA® Software for control and monitoring.
- Bionet F2-30A Bioreactor (Fig. 2) with MARTA® Software for control and monitoring.
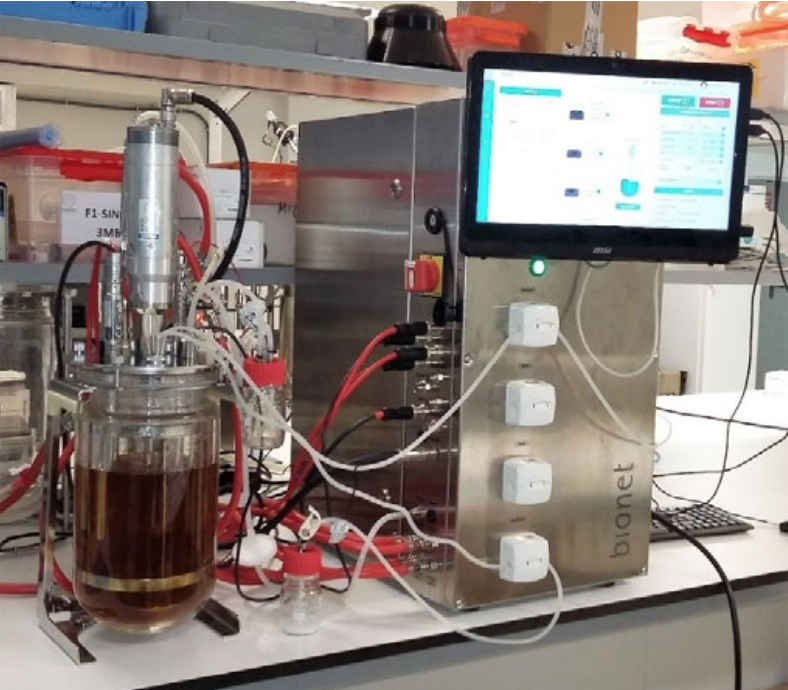

Fig2: Stainless steel Bionet F2-30A bioreactor used for scaling up, autonomous configuration (no steam installation required for sterilization). Available in models with working volumes of 15 and 30L, and in autonomous or non-autonomous configurations for sterilization.

Table 1: Comparison of Bionet bioreactor configurations for E. coli fermentation in fed-batch mode.
Media Unless otherwise stated, all reagents used in this work were purchased from Sigma-Aldrich (St. Louis, MO, USA) or Scharlab (Barcelona, Spain).
Inoculum
- Luria-Bertani (LB) Broth
- Terrific Broth (TB)
- Glucose (GLU)
- Ampicillin (antibiotic)
- Isopropyl-β-D-thiogalactopyranoside (IPTG)
- MgSO₄·7H₂O
- NH₄OH and H₃PO₄
- Sigma 204 Antifoam
Recombinant E. coli BL21 strains from a working cell bank (WCB) were propagated in 250 ml flasks containing 50 ml LB broth, 50 μg·mL⁻¹ ampicillin, and 4 g·L⁻¹ glucose for 12 hours at 37°C and 250 rpm in an orbital shaker until reaching an OD₆₀₀nm between 0.6 and 0.9 for inoculation into the 3-liter bioreactor. The inoculum ratio across all stages and scales was 1% v/v. Thus, for the equivalent operation in the 30-liter fermentation process, WCB stock flasks were propagated in 1000 ml flasks containing 200 ml LB, 50 μg·mL⁻¹ ampicillin, and 4 g·L⁻¹ glucose.
Fermentation
Fermentation assays were conducted in two separate experiments:
i. Characterization and optimization at the laboratory scale
• Batch mode to calculate the specific growth rate (μ) of the strain.
• Fed-batch mode using optimal feeding rate control.
ii. Scale-up from 3 liters to 30 liters
• Fed-batch mode using optimal feeding rate control based on bench scale characterization, and under the criterion of constant oxygen transfer coefficient (kLa).
For all experiments, inoculum cells in LB medium were propagated in Bionet bioreactors, containing 65% of the final fermentation volume at the start of the batch phase. The initial medium composition was 47 g·L^-1 of Terrific broth (TB), supplemented with 10 g·L^-1 of glucose, and 0.5 g·L^-1 of antifoam. Before inoculating the cells, the bioreactors were sterilized with the medium for 30 minutes at 121°C, using an autoclave for the glass bioreactor (Bionet F1-3MB) and steam-in-place (SIP) sterilization for the stainless-steel bioreactor (Bionet F2-30A). The glucose was autoclaved separately and added to the medium after the bioreactor sterilization. 50 mg·L^-1 of ampicillin as an antibiotic was added after sterilization and just before inoculating the cells. Throughout all experiments, the pH was maintained at 7±0.2 by adding NH4OH or H3PO4, the temperature was kept at 37°C during the first phase and 30°C during the protein expression phase, and dissolved oxygen was maintained at 30±1% saturation by regulating agitation and aeration rates with DO2 cascade control.
Batch mode in 3 liters
Cultures in the Bionet F1-3MB bioreactor were grown until reaching an OD600 nm of 0.6-0.9, after which IPTG was added to a final concentration of 0.5 mM to induce recombinant protein expression. Incubation continued for 8 hours for the production of recombinant protein. Optical density was monitored online with a turbidity sensor connected to the bioreactor and integrated into the ROSITA® control software.
Fed-batch mode in 3 liters
The first step before induction was equivalent to the batch mode with an OD600 nm of 0.9, with IPTG added to 0.5 mM as the final concentration for the induction of recombinant protein expression. Then, when dissolved oxygen increased to a value above 90%, the fed-batch mode was automatically started, activating a variable-speed pump to add a pre-sterilized glucose solution of 100 g·L^-1 containing MgSO4·7H2O at 18.7 g·L^-1. This solution was previously sterilized by microfiltration with a 0.2 μm pore size, starting with an initial feeding rate and increasing exponentially with the software’s exponential feeding function (Fig. 2), according to the simplified version of the exponential feeding equation [3]. The specific growth rate was controlled to 0.2 h^-1. Glucose feeding was maintained until an OD600 nm of 80±10 was reached:

⁃ Q(t): Feed solution flow rate (L·h^-1)
⁃ Xo: Initial cell density in g·L^-1 of dry cell weight (DCW)
⁃ Vo: Initial volume (L)
⁃ μ: Specific growth rate (h^-1)
⁃ Y·xs: Biomass yield coefficient (gDCW/g·glucose)
⁃ So: Substrate concentration in the feed solution (g·L^-1)
⁃ t: Time (h)
Scale-up from 3 liters to 30 liters
In this study, scale-up was applied under the criterion of constant volumetric mass transfer coefficient KLa, with a combination of a constant tip speed range. KLa was calculated using the physical dynamic method [4], according to the equation for determining the oxygen transfer rate (OTR).

Table 2: Summary of fermentation process scale-up criteria used in this study.
Centrifugation
To calculate the final biomass yield on glucose as Yx/s, a sample of the collected broth was centrifuged at 4500 × g at room temperature (RT) for 15 minutes using an OrtoAlresa centrifuge model Dicigen 21, with rotor RT 138. The resulting pellet was dried at 100°C for 1 hour to measure the dry cell weight (DCW).
RESULTS
Bench Scale Fermentation
Cell growth data were collected for process analysis from a CSV file generated by Bionet’s ROSITA® software for the bench scale. The growth rate (μ) in batch mode with glucose as the limiting substrate was calculated from the growth curve plotted on a logarithmic scale (Fig. 3). The specific growth rate obtained for this strain with glucose in the logarithmic phase was μ = 0.46h−1.

The optimal feeding rate conditions for the fed-batch strategy were established 40% below the maximum specific growth rate calculated in batch mode to ensure complete glucose consumption. When the culture altered the DO2 trend, indicating the complete exhaustion of the limiting substrate 3 hours after induction, the addition of the concentrated glucose solution was automatically initiated from the recipe programmed into Bionet’s software.
The final biomass concentration in fed-batch mode was approximately 25 g·L^-1 of dry cell weight (DCW), and the biomass yield coefficient YX/S was 0.63 g/g·glucose. These results were similar to those reported by other authors [5].
Scale-up to 30 Liters
The most common criteria for scaling up from laboratories to pilot scale and from pilot scale to production or plant scale are to keep one or more parameters similar between different scales. It is generally impossible to maintain all parameters in the same proportion relative to each other. These parameters include agitator tip speed, oxygen transfer rate (OTR), oxygen mass transfer coefficient (kLa), power per unit volume of the bioreactor, mixing time, and the Reynolds number of the agitator. Constant DO2 concentration is also used as a scale-up parameter for recombinant protein production [6]. For aerobic fermentation scale-up, the effect of gas-liquid mass transfer is the most significant factor. The tip speed criterion has some advantages in biotechnological processes involving microorganisms sensitive to the shear stress produced by an agitator, as it determines the maximum shear stress in the tank, which can cause cell damage [2]. Table 3 shows a comparison of the tip speeds calculated for Bionet F1-3MB and F2-30 bioreactors at different agitation rates (N), correlated according to the agitator diameter (D).

Table 3: Correlation between tip speeds (m/s) and agitation speeds (rpm) for the two scales.
An interval of KLa’s was calculated using the physical dynamic method [4], according to the equation for determining the oxygen transfer rate (OTR), for a minimum and maximum tip speed of 1.41 m/s and 4.24 m/s, respectively, with an airflow rate in the range of 0.5-1.5 vvm and a temperature of 37°C, using the medium without microorganisms (Fig. 4). The process was established to maintain a KLa value in the range of 50-120 h^-1 by varying agitation with DO2 cascade control, with an equivalent agitator tip speed in the range of 1.41-3.39 m/s and keeping the airflow rate constant at 1 vvm. As a reference value, preliminary characterization of the Bionet F1-3MB bioreactor determined a maximum KLa value of 300 h^-1, corresponding to the equipment’s maximum OTR of approximately 350 mmol·O2·L^-1·h^-1 (unpublished data).

Fig. 4: Volumetric mass transfer coefficient (KLa) as a function of agitator speed at 37°C with the medium used and different airflow rates in Bionet bioreactors (A) F1-3MB and (B) F2-30A.
On average, E. coli cultures with an OD600nm of 75 and a DCW of 25 g/L were achieved in both scales over 20 hours of the process, as shown in Figure 5. A brief description of the process parameter setup is presented in the recipe outlined in Table 4 for both scales.

Fig. 5: Comparison of the temporal evolution of online optical density data exported from Bionet’s ROSITA® and MARTA® software for Bionet F1-3MB and F2-30A bioreactors, respectively, from the scale-up trials in fed-batch mode.

Table 4: Recipe setup for E. coli fermentation process in fed-batch mode using Bionet bioreactors.
Conclusions:
High cell density in the E. coli process was achieved using fed-batch mode in the Bionet F1 Bench-top bioreactor and scale-up to the pilot bioreactor F2-30, with critical process steps automated through ROSITA® and MARTA® software, respectively. The strain was well characterized in batch mode by collecting online data and simplifying growth calculations to obtain the specific growth rate. By applying this growth rate ideally as an exponential feeding rate in fed-batch mode, a biomass yield 7 times higher than in batch mode was obtained. Successful 10-fold scale-up was achieved in the Bionet F2-30 bioreactor. In addition to a well-characterized strain and well-defined scale-up criteria, such as maintaining constant KLa at both scales, the ability of ROSITA® and MARTA® software to program specific exponential feeding rates at each scale was vital to the process success. Both platforms allowed us to obtain similar results across the scale-up and to collect valuable online and batch-end data for future process optimizations, such as increasing the total process time after induction to allow for a higher DCW and optimal expression of the protein of interest.